Chapter 1
A new nuclear age is beginning, and with it a true understanding that we can no longer afford to ignore the potential of this powerful technology. The history of nuclear power provides a stark example of how the politics around key solutions to progress can become warped, ultimately resulting in less good outcomes.
Analysis by the International Energy Agency[_] and Intergovernmental Panel on Climate Change[_] suggests that rapid expansion of nuclear power is needed to meet global climate goals. In fact, evidence suggests baseload sources of energy like nuclear power facilitate integration of renewable sources and help deliver low-cost electricity systems.
Yet, from early promise and enthusiasm in the 1960s and 1970s, nuclear energy began to face considerable opposition from protestors worried about public health and environmental impacts. This was fuelled by understandable concern about accidents at Three Mile Island, Chernobyl and, most recently, Fukushima, driving public opposition and reducing governments’ commitment to nuclear power.
The reality is that nuclear energy is a safe form of energy, with significant benefits in terms of reducing emissions and creating balanced, low-cost energy systems. Public perception of the risk of nuclear power is not commensurate with the actual risk. In the entire history of nuclear energy, there have been only two major accidents (those at Chernobyl and Fukushima) and their effects, while serious, have been significantly over-estimated.
The result is that nuclear energy has never become the ubiquitous power source many had projected, with countries instead turning towards alternatives such as coal and gas.
The world is now paying a price for letting lingering concerns about safety and ideological opposition deter governments from harnessing a key solution to powering economies in a clean way: If the ambitious approach to nuclear deployment had continued, the world would have saved 28.9 gigatonnes (Gt) of carbon dioxide (CO2) since 1991. This is 3.1 per cent of the energy-related emissions in this period, about one year of energy-related emissions, or the equivalent of shutting down 903 coal-power plants (of 380 megawatts) for the entire period.
Last year, global energy-related emissions would have been 6 per cent lower, saving 2.1 Gt of CO2. This would be the same as taking about 460 million cars from the road for a year or removing the combined total 2023 emissions of Canada, South Korea, Australia and Mexico.
Political leaders aspiring to meet rising energy demands, reduce energy costs and provide security and growth now have a choice to make. Whether they choose to build nuclear is entirely within their gift. Many are showing their willingness to move past false alarm and ideology, making judgement based upon fact-based assessment of risk. And they are moving fast towards the future.
Chapter 2
Nuclear energy emerged as one of the transformative innovations of the 20th century. It began with the discovery of the atom’s structure, revealing immense energy within, and quickly advanced as scientists uncovered nuclear fission in the 1930s – a process that releases vast energy by splitting atomic nuclei. While this breakthrough initially fuelled the development of nuclear weapons during the second world war, it also revealed peaceful applications. This sparked a shift in focus after the war, catalysed by United States President Dwight Eisenhower’s 1953 “Atoms for Peace” speech, which promoted nuclear energy for civilian uses.
The ability to harness atomic energy introduced a new, massive-scale way to generate power. Unlike fossil fuels, which rely on chemical reactions, nuclear fission releases millions of times more energy from a small amount of fuel – offering an abundant, reliable energy source. In the post-war period, nuclear power was hailed as an innovation capable of meeting global energy demand, with governments supporting it as a path to national prestige, energy independence and economic growth.
The United Kingdom led the way, opening the first full-scale nuclear power station at Windscale in 1956, inspiring other nations to launch ambitious nuclear programmes. Global nuclear capacity grew rapidly, rising from less than 1 gigawatt (GW) in 1960 to 100 GW by the late 1970s. The 1973 oil crisis further accelerated nuclear investment, particularly in oil-dependent nations such as France and Japan, which sought to reduce reliance on volatile imports. France undertook an extensive programme, building 25 nuclear plants in just 15 years, while the US significantly expanded its nuclear programme, commissioning dozens of reactors throughout the 1960s and 1970s.
Many expected this growth to continue, positioning nuclear energy as a ubiquitous power source that would shape the future of global energy production. But history did not turn out that way.
Concerns around nuclear safety have existed since nuclear power stations first came online. In the 1960s and 70s, a significant anti-nuclear sentiment began to take shape, driven by growing public concerns over safety, radioactive-waste disposal and the potential for catastrophic accidents. Environmental activists, influenced by the rise of the environmental movement, highlighted the risks associated with radiation exposure and the long-term management of nuclear waste, which could remain hazardous for thousands of years. Additionally, the potential for nuclear materials to be diverted toward weapons and military uses amplified these fears, particularly in the context of the Cold War.
Public awareness and scepticism surged in 1979, when the film “The China Syndrome” was released, depicting a fictional near meltdown at a nuclear plant. The film’s narrative resonated with existing fears and significantly influenced public perception, particularly when the real-life incident at Three Mile Island in the US occurred just days after its premiere.[_] This event marked an important moment in the anti-nuclear movement, fuelling protests and leading to increased scrutiny over the safety and regulation of nuclear energy. The US, the world’s largest producer of nuclear power, did not commit to a new reactor for nearly three decades after this.[_] Just one year later, Sweden decided in a referendum to gradually phase out nuclear energy.[_]
Anti-nuclear sentiment reached a peak after the Chernobyl nuclear-power-station disaster in 1986. Its impact on the commitment to nuclear energy was considerable. Italy halted its nuclear-energy programme in 1987 following a public referendum spurred by Chernobyl, and other countries including Germany, the Netherlands, Belgium and Austria took steps to reduce their nuclear ambitions.
In the decades following Chernobyl, nuclear power experienced a complex period of both caution and resilience. Despite the shadow of Chernobyl, technological advancements and improved safety protocols aimed to rehabilitate the image of nuclear energy. The increasing awareness of the link between greenhouse-gas emissions from energy and climate change also sparked an increasing interest in nuclear power, and the notion of a “nuclear renaissance” gained popularity.[_] The 1990s and early 2000s saw nations such as France and the US modernise older reactors and invest in safer Generation (Gen) III reactor designs.[_] Countries in Asia saw nuclear energy as essential to their growing power demands and environmental objectives.
The Fukushima Daiichi nuclear disaster in 2011 reignited global concerns over nuclear safety. Triggered by a 9.0-magnitude earthquake and a tsunami, the power plant suffered from cooling-system failures, reactor meltdowns and significant radioactive releases. This shook public trust yet again, highlighting even the most advanced systems’ susceptibility to unforeseen events. In response, Japan gradually closed all of the country’s 54 nuclear reactors[_] and increased fossil-fuel imports instead.[_] Elsewhere, countries such as Germany and Belgium hastened their nuclear phase-out, while others, such as Switzerland and Spain, decided not to build new nuclear plants.[_] Thailand indefinitely postponed plans to build its first nuclear-energy reactors.
Of course, public perception has not been the only driver of nuclear power’s decline. The potential of harnessing cheap gas diverted investment into gas-fired generation in many countries in the 1980s and 90s;[_] furthermore, liberalisation of energy markets made capital-intensive projects like nuclear harder to finance.[_] Additionally, the rise of renewable-energy technologies such as wind, solar and hydroelectric power offered governments an attractive clean alternative to nuclear power.
But the cost of nuclear is closely tied to the regulatory regime in which the technology operates and the amount of litigation the project is subject to. Over the years, countries have gradually introduced increasingly stringent safety and environmental regulations in response to public concern, with focus shifting away from innovation and improvement toward risk avoidance. Furthermore, the heightened concern led to a rise in litigation, as local communities and environmental groups frequently challenged the development of new nuclear facilities. These legal battles often delayed projects for years or even resulted in their cancellation. This, combined with a lack of clear commitment to pipelines of reactors, has inflated the cost of nuclear reactors over time, further creating a negative spiral for the technology.
Nuclear costs have risen over time in countries like the UK, US, France and Finland but remained low in South Korea
Source: Britain Remade
The result has been a considerable slowdown of nuclear-power generation. In the 32 years before Chernobyl, 409 reactors were opened; in the three decades following the disaster, only 194 were connected.[_] By the mid-1980s nuclear power made up about 15 per cent of global electricity generation; today nuclear power plants generate approximately 10 per cent of global electricity, sourced from about 440 reactors worldwide.
Nuclear-power generation began to slow in the 1990s
Source: Energy Institute, Statistical Review of World Energy
Chapter 3
The public understanding of nuclear power has been shaped by media headlines and dissemination of information around key accidents like Chernobyl, as well as the claims of the anti-nuclear movement. This has resulted in an inaccurate perception of the technology’s real risks and rewards.
There are chiefly three nuclear incidents that have shaped public opinion on nuclear: Chernobyl, Fukushima and Three Mile Island. There’s no denying each of these were serious accidents, but the public perception of what happened, and the knock-on impact it has had on the public’s perception of nuclear safety, is not completely in line with the reality.
Chernobyl
The Chernobyl accident took place on 26 April 1986 at the Chernobyl nuclear power plant located in northern Ukraine, then part of the Soviet Union. During a late-night safety test on Reactor 4, a combination of design flaws in the reactor and operator errors led to a rapid, uncontrollable power surge. This surge caused a series of explosions that ruptured the reactor vessel, releasing massive amounts of radioactive material into the atmosphere. Unlike more modern reactors, Chernobyl’s high-power channel reactor, called an RBMK reactor, lacked a containment structure, allowing radioactive particles to disperse widely across Europe. The area surrounding Chernobyl was contaminated, resulting in the creation of an exclusion zone and the displacement of hundreds of thousands of people. Soviet authorities initially attempted to downplay the disaster, delaying evacuation and exposing local populations to dangerous levels of radiation.
The media’s initial coverage of the Chernobyl disaster was marked by considerable alarm and speculation, especially in Western outlets. Following a two-day delay from Soviet authorities, who only vaguely acknowledged an “incident”, Western media quickly filled the information gap with dramatic headlines and worst-case-scenario analyses. Reports warned of “radioactive clouds” drifting across Europe, speculated about massive potential casualties and likened the fallout to nuclear warfare. The uncertainty, combined with alarming language and imagery of invisible radiation spread, stoked public fear across Europe and beyond, amplifying concerns about contamination and health risks.
The accident was a significant catastrophe. But research now shows that the effects were less severe than original reports feared. Our World in Data[_] has reviewed the evidence on the deaths resulting from the Chernobyl accident and found that, in total, two workers died on the scene, 28 people who had been on the scene died in the following weeks from acute radiation syndrome (ARS), and a further 19 survivors who suffered from ARS had died by 2006, most from unrelated causes. In addition to this, the United Nations Scientific Committee on the Effects of Atomic Radiation (UNSCEAR) found that between 1991 and 2015, 4,808 thyroid-cancer cases in patients under 18 in Ukraine, Belarus and exposed regions of Russia could be linked to radiation exposure as a result of drinking fresh milk containing radioactive iodine from cows that had eaten contaminated grass.[_] It was reported in 2005 that 15 of these cases were fatal. Our World in Data calculates that over time this could increase to between 96 and 384 as more people could eventually die from the cancer.[_]
In the period after the accident, there was considerable and justifiable concern about the impact of radiation in the surrounding area and across Europe. However, evidence suggests there were no negative health impacts on the wider European public from the Chernobyl accident. UNSCEAR concluded in its report that the average effective doses of radiation from Chernobyl due to both external and internal exposures between 1986 and 2005 were about 31 millisieverts (mSv) for the evacuees, 9 mSv in the contaminated areas, 1.3 mSv for residents of the former Soviet Union and 0.3 mSv for the populations of the rest of Europe.[_] The World Health Organization (WHO) has similarly found that exposure was 30 mSv for evacuees, 9 mSv in the contaminated areas and less than 1 mSv in the first year after the accident in other European countries.[_] To compare, the dose of a medical CT scan can be up to about 10 mSv[_] and the total worldwide average effective dose from natural background radiation is around 2.4 mSv per year.[_]
Several environmental groups claim that the true death toll from Chernobyl is closer to 100,000.[_],[_] This is not accurate. The UN has said that about 50 deaths can be directly attributed to the Chernobyl accident, and that 4,000 people could eventually die from radiation exposure.[_]
The Chernobyl disaster was largely a result of fundamental flaws in the Soviet-designed RBMK reactor and a lack of proper safety culture within the Soviet nuclear industry. The reactor’s inherent instability, especially at low power, combined with the absence of a containment structure, made it particularly vulnerable to catastrophic failure. Furthermore, inadequate training of personnel, poor operational procedures and a general disregard for safety protocols exacerbated the situation.[_],[_] The Soviet authorities’ initial attempts to downplay the accident and their delayed response in evacuating nearby populations further compounded the impact of the disaster. The Chernobyl disaster serves as a stark reminder of the consequences of poor design and management. However, over the years, significant advancements in nuclear safety protocols, reactor design and regulatory oversight make it less likely that such a catastrophic event will occur again.
Fukushima Daiichi
On 11 March 2011, a 9.0-magnitude earthquake occurred off the east coast of Japan. This generated a large tsunami that caused the Fukushima Daiichi Nuclear Power Station (FDNPS), located along the shoreline, to lose its core cooling capacity. The result was a significant nuclear accident in which the reactor’s core was damaged and radioactive materials were released into the surrounding area.
In the wake of the Fukushima accident, media coverage quickly escalated, with headlines focusing on worst-case scenarios and drawing immediate comparisons to Chernobyl. Dramatic visuals of explosions at the reactor site and reports of radioactive plumes heightened public anxiety, often emphasising the potential for widespread contamination.
However, the health impact of the event is more nuanced than is often perceived. Again, Our World in Data has reviewed the evidence, finding that while 40 to 50 people were physically injured by the blast or radiation burns, no one died as a direct result of the disaster. In the 19 months after the accident, workers were exposed to average radiation levels of 12 mSv. Of the total workforce, about 35 per cent received doses of more than 10 mSv over the same period and 0.7 per cent received doses of more than 100 mSv.[_] The Japanese government announced in 2018 that one worker had since died from radiation-related cancer.[_]
In terms of the impact of radiation exposure on the local population, the WHO and UNSCEAR estimate that the average lifetime doses for adults in Fukushima were around 10 mSv or less. This was roughly two times as high for 1-year-old infants.[_] According to UNSCEAR, by 2021, the estimated average annual effective doses in non-evacuated areas of Fukushima Prefecture were less than 0.5 mSv. In evacuated communities where orders have been lifted, the average annual effective doses were generally less than 1 mSv. For context, the average annual dose from natural background radiation in Japan is approximately 2.2 mSv. In 2016, the WHO noted that there was a very low risk of increased cancer deaths in Japan.
The most significant health impact from Fukushima was not the radiation but rather the impacts of the evacuation. The WHO reported an increase in mortality among elderly people who were placed in temporary housing following the disaster. This population also experienced an increased risk of diabetes, mental-health problems and other non-communicable diseases, which were made worse by the lack of access to health care in the wake of the evacuation.[_] In September 2020, it was estimated that this had led to about 2,313 premature deaths.[_]
Three Mile Island
The Three Mile Island accident occurred on 28 March 1979 at the Three Mile Island Nuclear Generating Station near Harrisburg, Pennsylvania. It began with a mechanical or electrical failure in the plant’s secondary cooling circuit, which caused the primary reactor to overheat. Operator errors exacerbated the issue, leading to a partial meltdown of the reactor core in unit TMI-2.
Following the accident, roughly 2 million people that were in the area around the TMI-2 reactor during the accident were estimated to have received an additional average radiation dose of about 0.01 mSv. To compare, the area’s natural radioactive background dose is between 1 to 1.25 mSv per year. The maximum dose a person would have received from the accident was less than 1 mSv above the background dose.[_] While questions were raised in the months following the accident about possible adverse effects from radiation on human, animal and plant life, none could be directly correlated to the accident. Columbia University and the University of Pittsburgh concluded the release had negligible impacts on the physical health of individuals or the surrounding environment.[_]
Chapter 4
Having investigated the actual consequences of these three accidents, the evidence strongly suggests that public perception of the risks of nuclear energy is not aligned with reality.
It is important to be honest about the drawbacks associated with nuclear power: it is not a renewable source of energy; it involves the need for management of radioactive waste, which carries additional risks and costs; and it is not possible to preclude the possibility of accidents. It is also highly capital intensive, which increases the risk of cost-overruns and delays. But the public perception of the risks is often not aligned with the real risks and problems with nuclear power.
Taking the key concerns often levied against nuclear energy one by one creates a far more nuanced picture.
There are trade-offs with nuclear power, just like there are trade-offs with any other energy-generation technology. But unlike other technologies, nuclear energy faces an enhanced, unfounded perception of risk, with far less consideration of the rewards.
A common claim from anti-nuclear activists is that no level of radiation is safe, which means that any radiation will always have a negative impact on public health and the broader environment. As the possibility of radiation being emitted into nature, even with safer reactors, cannot be completely precluded, their conclusion is that the risk of nuclear is not worth its benefits.
This assessment misstates the relative weight of the risks and rewards.
A comparison with coal energy generation illustrates this. While a nuclear accident is very unlikely (possibly reduced to less than once in 1,000,000 reactor-years), the consequences are vast as can be seen by the human and economic impacts of Fukushima and Chernobyl. Coal has a higher death rate, pollutes the air and ultimately contributes significantly to degradation of the natural world by helping to accelerate climate change. In fact, living next to a coal power station subjects a person to greater doses of radiation than living next to a nuclear power station. Nuclear is therefore on paper less risky than coal, but because the risk looks different, the technology is treated in a completely different way.
The same applies to other risks in society. As Britain Remade’s Head of Policy Sam Dumitriu points out,[_] living next to a nuclear power station involves a one-in-a-million chance of dying from a nuclear accident or normal operation at a nuclear power station; meanwhile, driving in the UK entails a roughly 29-in-a-million chance of dying each year. Driving has significant benefits for the individual and the economy, as does nuclear power. But driving remains a risk that people are far more willing to expose themselves to compared to living near a nuclear power station.
The relatively limited risks associated with nuclear energy are manageable, but the benefits of harnessing nuclear power are significant. Nuclear power is carbon-free; it generates more energy per square metre of land use than any fossil fuel or renewable energy source;[_] and it could help stabilise grids and provide low-cost energy systems. Analysis by credible organisations such as the International Energy Agency[_] and the Intergovernmental Panel on Climate Change[_] suggests that rapid expansion of nuclear power is needed to meet the world’s climate goals.
But excessive safety requirements make nuclear power less economical. For instance, the Office for Nuclear Regulation applies a principle that any safety measure is justified as long as its costs are less than ten times its benefits.[_] The practical effect of this approach can be seen with construction of reactors at Hinkley Point C. Even though it is based on a French reactor design, the ONR required a staggering 7,000 design modifications.[_] Hinkley Point C will now use 25 per cent more concrete and 35 per cent more steel than it would otherwise.[_] These types of bespoke changes drive up project costs, increase the time and resources to get approval and build the reactors, and limit the possibility of learning rates from similar projects. The outcome is that Hinkley Point C is one of the world’s most expensive reactors. Similar situations are seen in other countries.
Similar principles are applied elsewhere, with similar results. At the Vogtle nuclear-power-plant expansion in Georgia, in the US, a number of design changes to the AP1000 design resulted in huge project delays and cost overruns. The total cost increased from an initial estimate of $14 billion to around $34 billion to $35 billion.[_] The Olkiluoto 3 nuclear power plant in Finland, originally estimated to cost €3 billion and be completed by 2009, faced significant delays and cost overruns, ultimately taking 18 years to construct and costing approximately €11 billion by the time it began commercial operation in 2023,[_] all due to requirements for bespoke changes driven by stringent standards for safety and environmental protection.
To tackle climate change, and in particular to deliver nuclear energy at scale, countries globally need a better understanding of risk and how to balance it with reward.
Chapter 5
The world is paying a significant price for turning its back on nuclear energy. Counterfactual scenarios tell the story.
Growth rate for nuclear year on year was between 30 and 40 per cent in the 1960s, 20 to 25 per cent in the 1970s, 10 to 15 per cent before Chernobyl in the 1980s, and dropped to around 1 to 2 per cent in the 1990s, 2000s and 2010s. This steep decline after the 1980s was not a given.
To explore what could have happened if the world had not turned away from nuclear power, we have segmented the world into three groups:
High nuclear capacity: Countries that had already started adopting nuclear technology or were in a position to do so before Chernobyl, including European countries, countries in the former USSR, countries in North America, Japan and South Korea.
Medium nuclear capacity: Countries that were beginning to develop rapidly in the period following Chernobyl and that could have capacity to begin to develop nuclear programmes, but mostly had not done so before 1986. This includes China, India, Indonesia, Vietnam, Brazil and Argentina, among others.
Low nuclear capacity: Developing countries that were unlikely to develop nuclear programmes due to the high capital cost and poorly developed grids.
For countries with high nuclear capacity, we assume that rate of nuclear as a share of electricity generation rises in line with observed rates for the first five years after Chernobyl (1986 to 1991) and then continues to rise at the same average rate as observed in these five years (2.75 per cent) every year until it hits a ceiling of an average of 33 per cent of total electricity generation. For comparison, the actual average was 16.6 per cent in 2023.
Assuming that nuclear would only displace fossil fuels, given its similar role as a baseload source of power, this would mean that energy-related emissions in this group of countries would have been 10 per cent lower in 2023. Over the whole period, these countries would have saved 4.8 per cent of emissions, equalling 1.6 years of energy-related emissions. This is 24.2 gigatonnes (Gt) of CO2.
Counterfactual carbon savings for countries with high nuclear capacity
Source: Energy Institute, TBI
For countries with medium capacity, we assume that nuclear as a share of electricity generation follows observed rates until 1996 when it begins to increase by 15 per cent every year ending at 11 per cent. This assumes China in particular had a more aggressive nuclear adoption. The actual average growth rate in this period was 7 per cent.
Emissions in this group would have been 4.75 per cent lower in 2023 and 1.4 per cent lower over the whole period, equalling slightly less than half a year of this group’s energy-related emissions. This is 4.7 Gt of CO2.
Counterfactual carbon savings for countries with medium nuclear capacity
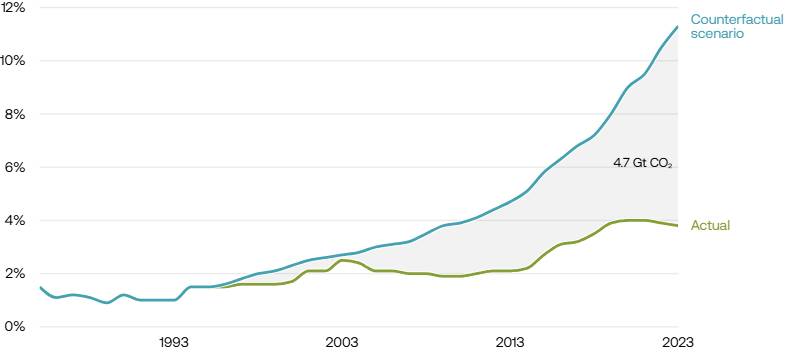
Source: Energy Institute, TBI
For the final group of countries, we have assumed no change in nuclear uptake.
Taken together, this means that the world as a whole would have saved 28.9 Gt of CO2 since 1991. This is 3.1 per cent of the emissions in this period, about one year of energy-related emissions, or the equivalent of shutting down 903 coal-power plants (of 380 megawatts) for the entire period.
In this scenario, global energy-related emissions in 2023 would have been 6 per cent lower, saving 2.1 Gt of CO2. This is the same as taking 460 million cars from the road for a year. It would be the same as removing the combined 2023 emissions of Canada, South Korea, Australia and Mexico.
These strong projections of emissions savings today based on observed rates of nuclear uptake in the early 1990s could still be an underestimate. In the 1990s and 2000s, countries’ decision-making around energy technologies began to shift from being simply a question of cost to incorporating questions around climate impact. If the perception of nuclear had remained more fact-based, nuclear energy could have become an even more attractive alternative and the result could have been a stronger global move towards nuclear, coupled with renewables, for baseload-power provision.
This shows the significant impact that turning away from nuclear has had on our ability to tackle climate change. But there are also likely wider implications – higher penetration of nuclear energy could help increase countries’ energy independence and insulate them from fossil-fuel shocks. It could help deliver low-cost electricity and improve the conditions for renewables integration in lieu of coal or gas.
These effects can be seen in the countries that shunned nuclear power.
Japan – which shut down all its nuclear reactors after Fukushima – has ended up with the dirtiest grid in the G7 and paid $510 million a day for fossil-fuel imports last year.[_] If the country had maintained nuclear capacity at the same level as before Fukushima, its energy-related emissions would have been 14 per cent lower in 2023. Since 2011, the country would have saved 143 megatonnes (Mt) of emissions – about 14.1 per cent of its energy-related emissions in the period.
Similarly, in Germany, the fall in nuclear power has offset more than 70 per cent of the energy added from renewables. If its nuclear capacity had been maintained at 2002 levels, energy-related emissions would have been 21 per cent lower in 2023, and the country could have saved 1.1 Gt of emissions since 2002 (a bit more than 3 per cent of its emissions in this period). This would mean that Germany would have reduced its emissions by 55.6 per cent on 1990 levels, putting the country ahead of schedule for delivering its target of 65 per cent reduction of emissions on 1990s levels by 2030. It would also have resulted in lower energy costs and enhanced grid stability.[_]
Even the United States, which maintained its nuclear fleet but scaled back expansion after the Three Mile Island incident, could have saved significant emissions in this period. Before the 1980s, the US was following a similar path to France in terms of nuclear uptake. Assuming nuclear commitment had remained high, and that nuclear generation reached 40 per cent and was maintained at that level, the US would have saved 5.3 Gt of emissions since 1985, or 2.6 per cent of its total energy-related emissions in this period. In 2023, US energy-related emissions would have been 2.9 per cent lower, saving 135 Mt of emissions.
France, on the other hand, maintained a strong commitment to nuclear, resulting in one of the lowest carbon footprints in Europe. This has enhanced its energy security by reducing dependence on imported fossil fuels and stabilising electricity prices due to predictable generation costs. As the world’s largest net exporter of electricity, France earns more than €3 billion annually from exports and enjoys low generation costs. Its advanced nuclear infrastructure has also established the country as a global leader in nuclear technology.[_]
Chapter 6
The world is now yet again turning towards nuclear. Last year at COP28, 25 countries came together and committed to tripling global nuclear capacity by 2050. This commitment has been backed up by pledges from some of the world’s biggest banks and financial institutions to increase their support for nuclear energy. In the high-case scenario of the International Atomic Energy Agency outlook, nuclear capacity is projected to more than double by 2050, a figure that was recently adjusted upwards by a quarter since 2020. It is not unlikely that this projection will be increased again.
Several trends are steering the world back towards nuclear. Energy shortages in the wake of the pandemic recovery and the Russian invasion of Ukraine have shifted public and elite opinion towards nuclear as a source of clean, secure energy. Large AI hyperscalers, which depend on reliable low-carbon baseload power, are pouring money into new nuclear projects. And new innovations in nuclear technology are emerging that encourage wider use of the technology for data centres and industrial processes. This all creates considerable global momentum behind nuclear.
This growing momentum can be seen around the world. Currently about 60 reactors are under construction globally, with 110 more planned and more than 300 proposed.[_] This compares to a current fleet of about 440 operational reactors. Most of the new reactors are in Asia, with China leading the way having added 34 GW of nuclear-power capacity over the past ten years;[_] an additional 27 reactors are under construction;[_] and there are plans to build 150 new reactors between 2020 and 2035.[_] This is a rapid expansion, and while the US still lays claim to having the largest nuclear fleet at 94 reactors, it took the country nearly 40 years to build the same nuclear-power capacity as China added in ten years.[_]
Other countries are turning to nuclear too. India has a goal of tripling nuclear capacity to 22 GW by 2032, and further growing capacity to 100 GW by 2047, as a part of its “Developed India” strategy. The US recently published a roadmap for tripling its nuclear capacity to respond to load growth. Countries in Europe that rely heavily on coal, such as Poland and the Czech Republic, have been showing increasing commitment to nuclear power, including designating several sites for new small modular reactors.
Some of the most recent announcements for new nuclear have been highly symbolic. The US is re-opening Three Mile Island Unit 1 – the counterpart of Unit 2, whose partial meltdown fuelled so much nuclear opposition. Japan is also increasingly recommitting to nuclear, even re-opening reactors near Fukushima on parts of the coast that survived the impact of the 2011 tsunami.[_]
The public perception of nuclear energy is also improving rapidly. In the UK, YouGov polling shows that a majority of the public now thinks the government is right to spend money on supporting new nuclear, with a majority having opposed it as recently as 2021.[_] It is now the second most supported type of energy generation.[_] Similar positive trends in public support can be seen in countries like the US.[_] Younger people are also more pro-nuclear,[_] further illustrating that the long shadow of accidents is being replaced by a sense of urgency for addressing climate change.
In other words, a new nuclear era is beginning. But whether it continues will depend entirely on whether governments can better handle the risks and public opinion. Only then will they harness the power of nuclear quickly and at low cost.
Chapter 7
This is a pivotal moment in the fight against climate change. Accelerated action is needed in every country across the world, with more rapid deployment of all types of clean technologies and new solutions to deliver clean power for all.
To achieve this, the world must learn the lessons from the history of nuclear energy. Whether it is the new nuclear renaissance or other technologies that will help fight climate change, the world cannot afford to let unfounded public concern to stand in the way of progress.